A Liquid Oxygen Release – Should I Be Worried?
Andrew Byrnes
Interviewing dozens of experienced hazmat instructors and students I found some interesting opinions regarding a Liquid Oxygen (LOX) response. The standard precautions taken by most responders to ensure safety are: 1) do not step on frozen asphalt, it could explode, 2) do not drive over frozen asphalt, the pressure could detonate under the weight of the vehicle, 3) do not allow LOX to come into contact with combustible materials, such as dried grass in the median, or hydrocarbons, such as diesel fuel, because it will spontaneously ignite resulting in a fast and intense fire and, 4) wait 30 minutes after the last frost is gone before stepping on the asphalt.
I’ve taught these precautions over the years myself, trusting what I have always been taught. The chemistry side of my brain said – “wait a minute”. What is providing ignition? I have fuel, and I have oxygen, but where is the energy of the ignition? Have these precautions ever been scientifically tested and verified? Understanding that oxygen is a powerful oxidizer, would it really “ignite” hydrocarbons by simply contacting them? I asked myself if it could really happen. I decided it was time to test the “standard” precautions related to LOX response.
I spent hours researching books, articles, and research papers in any way related to LOX. The sum of the precautions and “facts” I found regarding LOX were unsubstantiated and not independently verifiable. Most of the research was anecdotal and like Martel’s Chemical Risk Analysis, “George Claude was seriously injured in 1903 after inserting a candle into liquid oxygen” (2000, pg. 242), or from the National Fire Protection Association (NFPA) 53, Annex D, which lists 63 types of LOX incidents but with the caveat, “NFPA cannot guarantee the accuracy of the reports” (NFPA 53, D.1.3). None of the 63 “incidents” in NFPA 53 Annex D were corroborated. The Compressed Gas Association pamphlet 2.7 on the handling and use of LOX systems in healthcare facilities states, “Stepping on or rolling equipment across a liquid oxygen spill can result in explosive ignition of combustibles.” (CGA, 4.1.2.7).
In my research efforts, I could not find a verifiable incident in the nation. Regardless, our testing at Utah Valley University (UVU) showed that when LOX saturates a combustible material and then an ignition source is introduced – violent and vigorous combustion occurs with increased burn rates, light, and heat. Without an ignition source or when ignition sources were controlled, LOX did not pose a significant hazard beyond those associated with cryogenic liquids.
We subjected LOX frozen asphalt samples to a series of impact tests by raising an object to a specified height and dropping it onto the sample. We used a weighted rubber fire boot to step and stomp on a frozen sample. We then struck samples with a 10 lb. sledgehammer, dropped a halligan (blunt headfirst), pipe wrench (headfirst), pike pole (point first), screwdriver (point first), and drove a fire engine over a larger conditioned asphalt surface and liquid pool of LOX. Forces were determined by subjecting a force plate device to these objects and digitally measuring the results of the force and calculating the height, pressure, and energy of the drop. None of the sources of mechanical impact or pressure caused any reaction in the asphalt after a minimum of five tests.
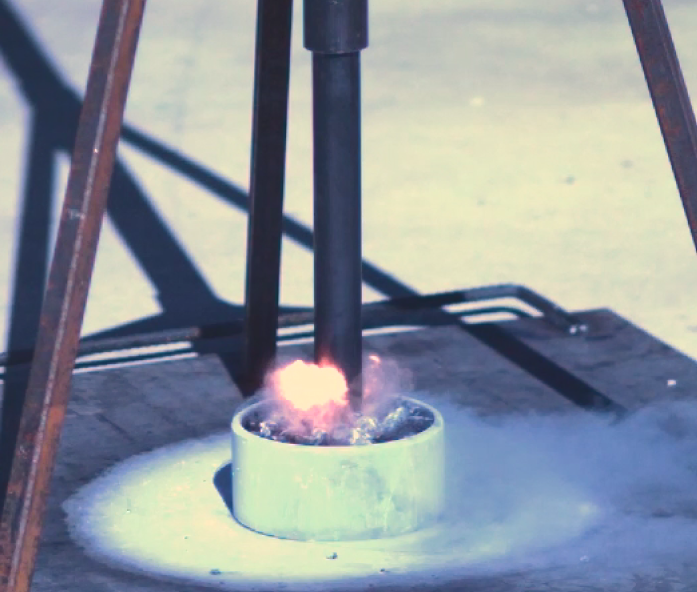
NASA, in 1973, impact tested LOX and crumbled asphalt as runway material. To everyone’s surprise, the LOX-soaked asphalt detonated and blew the NASA apparatus 30 meters into the air and created a debris field 50 meters in diameter (Moyers, Bryan, & Lockhart, 1973, p.11). At UVU, we wanted to replicate and verify NASA’s 1973 test using a scientific method. We were successful in replicating the NASA test, and so could endorse and validate their results. Using the American Society of Testing and Materials (ASTM) Standard Test Method for Determining Ignition Sensitivity of Materials to Mechanical Impact in Ambient Liquid Oxygen and Pressurized Liquid and Gaseous Oxygen Environments (G86-17), the configuration of drop mechanisms used to test the sensitivity of materials to mechanical impact pressure was built at UVU in compliance with the ASTM standard. (Figure 1).
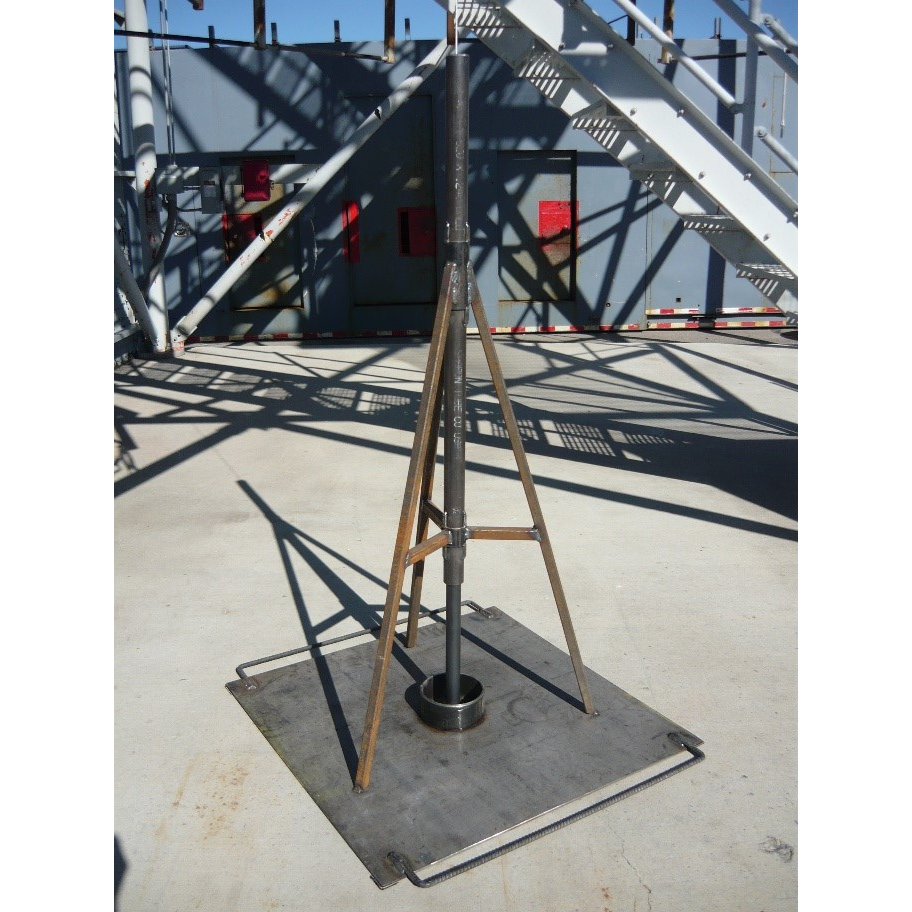
The ASTM states that any one reaction in 20 drops indicates the tested material is “reactive.” We experienced five reactions in 20 drops and were able to duplicate those reactions again 60 days later.
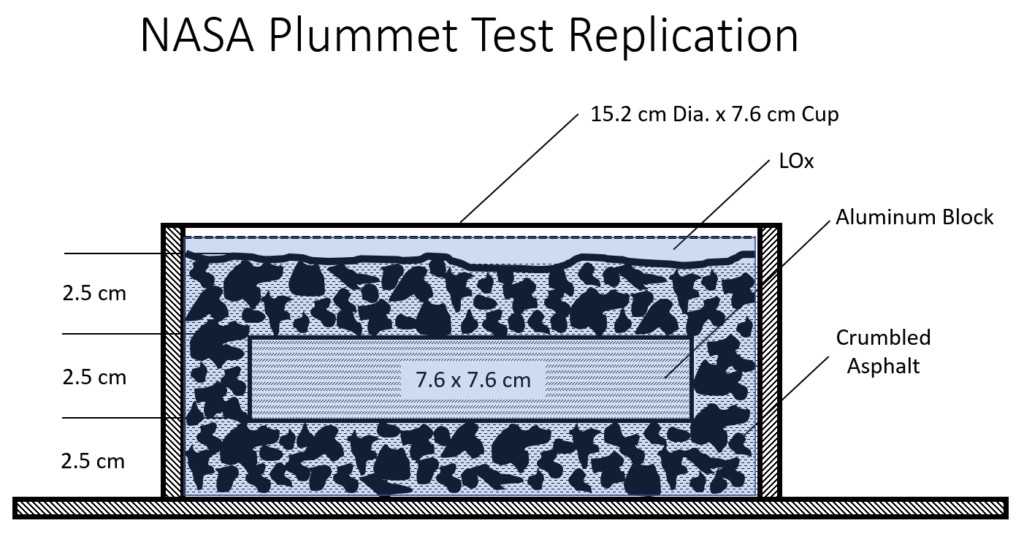
(Figure 2). The tested strata, used by NASA and UVU, were composed of crumbled asphalt, on which an aluminum block was placed, then covered by additional crumbled asphalt before being immersed in LOX. (Figure 3). Testing other configurations resulted in no reactions. Our highways do not consist of crumbled asphalt covered with a one-inch-thick plate of aluminum which is then covered with more crumbled asphalt. These reactions should be viewed with that important context.
In real time, we knew that a reaction had occurred when we heard what sounded like a gunshot. The subsequent flash was too quick for the naked eye. Chemistry and physics faculty at UVU in consultation with Eugene Ngai, a compressed gas expert, explained the likely ignition source. The reaction occurred as the plummet compressed the micro-bubbles in the LOX against the aluminum, almost instantaneously increasing the pressure and temperature of the oxygen gas inside the bubble, then releasing that energy as adiabatic heat. The adiabatic heat principle is like what occurs in the cylinder of a diesel engine due to compression.
To determine non-impact reactivity, we poured LOX directly onto an asphalt surface and dropped a road flare into the LOX pool. Other than some increased flare length and burning of the flare paper, no flaming combustion or explosion occurred. We poured LOX directly into 11 different, commonly available, hydrocarbon compounds and observed no reaction other than creating a frozen liquid. Saturated and unsaturated hydrocarbons, synthetic and natural compounds, alcohol-based products, various viscosities, and three liquids with flash points below 100° F did not react in contact with LOX. Likewise, combustible materials, such a cup of potato chips, oily and organic, did not react in contact with LOX. That said, when any of these combinations of hydrocarbon liquids or organic materials and LOX met with an ignition source, the combustion was rapid and intense. Combustion, influenced by LOX, is noticeably more rapid and vigorous than “normal” combustion occurring in our atmosphere of 21% oxygen.
The UVU tests also considered static electricity. We found a static spark to be an unreliable source of ignition. The spark was certainly hot enough (+1,800° F), however, the duration of the heat source, only milliseconds, may have been too brief to cause ignition. Arcing, caused by shorting out the positive and negative sides of a 12V battery, produced visible sparks and molten metal beads which immediately ignited any combustible fuel in LOX. Don’t discount the arcing of a vehicle battery short circuit on the scene when controlling ignition sources.
Conclusions:
- LOX soaked and frosted over asphalt will not react from the pressure associated with being stepped on, stomped on, driven over, or impacted by common response tools that are dropped on it, or the pressure from a direct sledgehammer strike.
- LOX, spilled on asphalt, would be extremely difficult to ignite with common ignition sources found on the emergency scene. Heat sources added to LOX/asphalt combinations only increased the rate of vaporization of the LOX.
- LOX will not react on contact with common combustibles, organic materials, flammable materials, flammable liquids, and other common hydrocarbons unless an ignition source is introduced – in which case the combustion will be violent and instantaneous.
- The NASA explosion from the plummet test in 1973 was successfully replicated at UVU however, circumstances leading to the detonation of the LOX and asphalt configuration are unlikely to be encountered, i.e., the aluminum plate inserted in a crumbled asphalt stratum. Explosions could not be replicated using solid or crumbled asphalt and LOX alone, a much more likely configuration.
- Practical testing of hazards associated with LOX should be expanded in the future.
Responders should take every precaution necessary when dealing with the primary hazards of LOX, namely embrittlement of surfaces in contact with the super-cooled liquid, high expansion ratios, and frost formations. Anytime LOX is mingled with combustible materials or flammable and combustible liquids, sources of ignition should be eliminated due to the possibility of extremely vigorous combustion. Any modifications to your agency’s procedures should be evaluated carefully based on these conclusions.
Website: https://www.uvu.edu/es/liquid_oxygen/index.html
References:
American Society of Testing and Materials. (2017). Standard Test Method for Determining Ignition Sensitivity of Materials to Mechanical Impact in Ambient Liquid Oxygen and Pressurized Liquid and Gaseous Oxygen Environments (G86-17). ASTM International.
Compressed Gas Association. (2018). Guideline for the safe storage, handling, and use of small portable liquid oxygen systems in health care facilities. 5th Ed. P-2.7-2018. CGA Inc.
Martel, B. (2000). Chemical risk analysis: A practical handbook. London: Penton Press.
Moyers, C., Bryan, C., & Lockhart, B. (1973, December). Test of LOX compatibility for asphalt and concrete runway materials. TM X-64086. National Aeronautics and Space Administration (NASA). Kennedy Space Center, FL.
National Fire Protection Association. (2021). NFPA 53: Recommended practice on materials, equipment, and systems used in oxygen-enriched atmospheres. NFPA. Quincy, MA.